Infusion Pump Performance: Flow Accuracy and Continuity Often Don’t Add Up
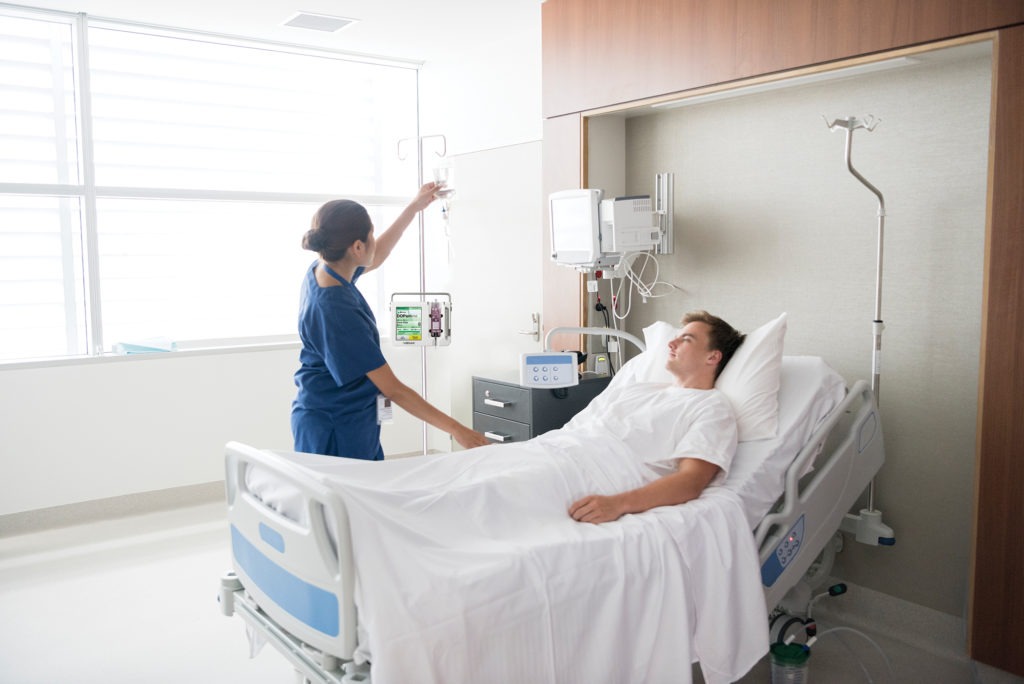
By Ben Powers and Ivyruth Andreica, PharmD, BSN, FISMP
Approximately 90% of hospitalized patients receive an infusion. Providers assume infusion pumps deliver medications as intended at an accurate and consistent flow rate. This is particularly important for high-alert medications, which pose significant risk when improperly delivered.
Pumps undergo extensive pre-market testing, and vendor manuals provide detailed documentation of the results. However, pump performance in the real world often differs significantly from lab results, with a potentially significant impact on patient safety.
Therefore, a fundamentally new paradigm in pump engineering is required to ensure high quality patient care and safety. An adaptive pumping system that automatically compensates for external factors impacting fluid flow will help administer infusions as the clinician intended. Currently, new technologies enabling this are under development, as recognition of flow problems and their potential patient harm grows.
Current State of Large Volume Infusion Pumps
Large volume infusion pumps (LVP) are assumed to infuse fluids and medications continuously at the programmed rate. Infusion pump user manuals typically report flow accuracy of +/-5%, but this is an average under controlled lab conditions (Weinger, Kline, 2016). Actual pump performance in real-world conditions typically is less understood, despite the dramatic effect changing conditions can have on pump output and patient response.
Fluid Dynamics: The Importance of Accuracy and Continuity
Knowledge about true LVP flow accuracy and continuity is vitally important for quality patient care. LVP flow accuracy is how closely the average pump flow rate correlates with the programmed rate. Typically manufacturer values are based on an average over a 60-minute interval. Flow continuity measures the variance from that average during the 60-minute window.
In LVP technical documentation, both accuracy and continuity are communicated using trumpet curves, as defined in the international standard for infusion devices: IEC 60601-2-24 (IEC, 2012). Flow rate during the first two hours of infusion is plotted in a startup graph. The first hour is considered warm-up time. Multiple calculations are performed during the second hour. The initial computation measures the overall flow accuracy and provides statistical analysis of flow variability during a range of time intervals. From those measurements, expected maximum and minimum variations are calculated. As the time interval for the averages increases, small fluctuations in flow rate are eventually smoothed and the variability decreases. These results are expressed in the trumpet curve.
Startup and trumpet curves showing computed pump performance data are illustrated in Figure 1.
Manufacturers typically report startup graphs and trumpet curves in their user manuals to provide approximate information for a specific LVP. However, trumpet curve testing in a controlled lab fails to account for many conditions potentially impacting pump performance in clinical settings.
The differences between real world accuracy and continuity can be illustrated by two hypothetical large volume pumps programmed to infuse at 90 mL/hr. Pump A delivers a continuous output of 80 mL/hr during a 60-minute infusion. Pump B alternates between delivering 180 mL/hr for 5 minutes followed by a 5-minute flow cessation, repeated cyclically during the 60-minute infusion. Pump A delivers 10 mL less than the target volume over an hour with an overall accuracy error of -11% over the hour (-10 mL / 90 mL = -11%), but has excellent flow continuity. Pump B averages a flow rate of 90 mL/hr since it is running at 180 for half the time and is inactive for the other half (180 mL/hr + 0 mL/hr) / 2 = 90 mL/hr). Thus, Pump B delivers the target 90 mL over the hour without any accuracy error, but has poor flow continuity. The performance of both pumps is illustrated in Figure 2.
Flow Characteristics in the Clinical Environment
In a real-world environment, various environmental factors may dramatically affect flow rates.
The Nature of Peristaltic Pumps
Currently most LVPs rely on peristaltic operation, typically using rollers to pinch down on a length of flexible tubing to move fluid forward (FDA, 2017). While there are variations in the exact mechanics, this general operation is common across most leading vendors.
Peristaltic fluid movement is fundamentally discontinuous, as various pump elements that propel and occlude fluid movement come into contact with the tubing.
At high infusion rates, pumping strokes might last seconds or minutes In these cases, flow rate variation is also of short duration and does not register noticeably in trumpet curve testing. With slower infusion rates when pumping stroke duration increases, flow variations become more visible in curve analysis. Most LVPs can deliver fluids at rates as low as 0.5 mL/hr (or below) with pumping strokes that may last several minutes to an hour.
Most systems incorporate a stepper motor to drive the peristaltic mechanism. Stepper motors move in discrete portions along the IV tubing leading to a finite but constant volume. At extremely low pumping stroke rates the time between drive motor actuations increases to seconds or even minutes. Similarly, to the Pump B scenario above, the result of this variance is short-duration flow rate spikes, followed by periods of little or no flow.
A sample startup graph and trumpet curve for a typical large volume peristaltic pump operating at a target flow rate of 0.5 mL/hr appear in Figure 3. The flow variation in the startup graph is indicative of a pumping stroke that repeats approximately every 20 minutes. The stroke initiates with a flow rate that is substantially higher than the target. The flow gradually decreases through the stroke and ends at a rate substantially below the target. The trumpet curve illustrates this, showing variability up to 50% during the 2-minute observation window. Variability remains high throughout the 5-minute observation window, showing deviations of ~25%.
Factors Impacting Accuracy in Real World Clinical Scenarios
The fluid container height relative to the LVP significantly affects accuracy. This position determines the fluid weight and pump pressure exerted at the tubing inlet. Along with tubing stiffness, this drives tubing fluid uptake during the fill portion of the pumping cycle. Published pump manufacturer’s guides indicate that variation due to changes in inlet pressure can add additional flow deviations of nearly 3%.
Likewise, outlet pressure variation causes changes to the volume of the flexible tubing. The IEC 60601-2-24 standard cites outlet pressure ranges of -100 mmHg to 300 mmHg due to variation in the orientation of the pump to the patient and the backpressure of small-bore catheters. Published numbers show high backpressures slow infusions by up to 9%, while low backpressures increase infusion rates by up to 7%.
Tubing material properties can also dramatically affect accuracy. For example, published data show tubing made of silicone remain effectively consistent over time. However, base tubing materials such as PVC are less resilient and eventually lose their ability to fully rebound. This reduces the volume contained in the tubing and decreases the average flow.
Many clinicians are unaware of these factors, which may result in infusion delivery inaccuracies of as much as +/- 30%.
The Potential Impact to Patient Safety
Approximately nine out of 10 hospitalized patients receive intravenous infusions (Baranowski, 1995). LVPs are used to deliver most intravenous fluids, especially high-alert medications. Medications with very short half-lives need continuous administration for therapeutic efficacy and outcomes. These medications often are administered to acutely ill patients to address blood pressure or arrhythmias, provide sedation, or in some cases for therapeutic paralysis. Clinicians should be aware of the clinical impact of the LVP used for delivery.
Discontinuous Flow
Norepinephrine is a mainstay therapy for hypotensive patients in septic shock. Norepinephrine has a half-life of approximately three minutes and a short duration of action of approximately two minutes. This means discontinuous delivery may then require a bolus (if appropriate), dose increase, or adjunct therapy. An LVP that continuously and consistently delivers the appropriate dosing, versus small bursts, may lead to beneficial patient outcomes.
Inaccurate Flow
Similarly, accurate delivery of Pitocin® (oxytocin), a high-alert synthetic hormone used for induction during labor, is paramount. Recommendations for oxytocin include administration at the lowest possible dose to achieve a positive effect using an LVP with accurate flow rate (American College of Obstetricians and Gynecologists, 2009). Quick delivery may lead clinicians to misjudge the drug’s full effect, leading to over-medication. Unrestricted pump flow poses another risk. Since oxytocin’s duration of action can last up to three hours, this can lead to prolonged, unnecessary side effects, such as uterine rupture and fetal deceleration. Such an infusion error involving an LVP currently on the market was reported to the FDA in 2017 (FDA, 2017, December).
Inaccurate flow of medications requiring precise delivery also can compromise patient care. For example, Blincyto® (blinatumomab), a monoclonal antibody used in the treatment of acute lymphoblastic leukemia, must follow specific timing protocols varying from 24 hours, 48 hours and seven days (Blincyto, 2017). Infusion delivered sooner than intended or infusing the dead space volume following a programmed delivery can result in adverse events.
These are not isolated examples and emphasize the need to investigate accurate medication delivery.
Raising Awareness
LVP manuals generally provide sufficient information to convey expected pump accuracy and flow continuity under many clinical scenarios. Trumpet curves are accurate. However, often the details fail to resonate with clinicians, who continue to expect +/- 5% accuracy and continuous flow under all conditions.
Users must become cognizant of deviations in LVP performance in the real world and compensate for them in patient care. The FDA and the infusion pump industry are aware of this disconnect. AAMI is currently working towards revised infusion device test standards aimed at more clearly conveying actual pump performance under various conditions and the potential impact on the patient.
This focus on more accurate and descriptive disclosure, however, misses a broader point. If clinicians are expecting better accuracy and continuous flow, why can’t LVPs deliver?
The Need for Adaptive Infusion Pump Technology
Enhanced pump performance across a full range of conditions requires a new paradigm in pump engineering. It calls for an adaptive technology that seamlessly interacts with the main elements of the traditional gravity-driven peristaltic pump – pressure (head height) and resistance (roller clamp) – to achieve continuous flow. Rather than propelling fluid with a fixed-displacement mechanism, it should directly apply pressure to push the fluid and provide a restriction in the flow path to regulate delivery. The result is greater accuracy and continuous fluid flow through the system.
Representative adaptive pumping system startup and trumpet curves illustrate the significance of the improvement. The startup curve below shows smooth, continuous flow, without the major cyclical deviations characteristic of fixed-displacement pumps, Figure 4. The smoother response is also clear in the trumpet curve, where variability stays within 5%, even when averaged over a 2-minute window.
Beyond Flow Continuity: Measure and Respond
While a pressure-based system will improve flow continuity, it also must address average pump output under changing external conditions. Backpressure in the tubing remains an important factor affecting flow rate. To compensate for this, adaptive pump technology must include a means to measure the actual fluid output from the system.
Existing peristaltic LVPs rely on pre-determined position or speed measurements of the various pumping elements. If, for example, a motor is turning at the proper speed, the system assumes that flow output is as desired. However, in reality, external conditions also influence the fluid path. For example, the motor might be moving correctly, but an increase in downward head pressure from the medication container may alter the fluid volume in the tubing. Currently, the impact of this is not measured and compensated for by infusion system software.
By contrast, a pneumatically-driven, adaptive pumping technology enables direct measurement of volumetric changes right at the point where fluid exits the pump and enters the patient. This direct flow measurement allows adjustment of the LVP through its control software to maintain target flow rates as external conditions change. Now, while an increase in backpressure might decrease flow, the system recognizes this and makes adjustments to bring the rate back to the target.
The combination of a smooth profile and an adaptive measurement and control system ensures that the pump is delivering what the clinician intended, regardless of system dynamics and external factors.
Conclusion
Most clinicians assume a +/- 5% accuracy and continuous flow under all conditions for their LVP. New test and disclosure standards focusing on accuracy and flow continuity are underway. But, more importantly, a new paradigm for fluid delivery is necessary. It must enable LVPs to adapt to external conditions while delivering fluids continuously and accurately. For now, clinicians should be aware of how today’s LVPs behave under real-world conditions and relate this to patient care.
References
American College of Obstetricians and Gynecologists. (2009, August). ACOG Practice Bulletin No. 107: Induction of Labor, Clinical Management Guidelines for Obstetrician-Gynecologists. Obstet Gynecol, 114, 7. Retrieved from https://www.mnhospitals.org/Portals/0/Documents/patientsafety/Perinatal/acog–practice_bulletin_107_2009.pdf
Baranowski, L. (1995). Presidential address: take ownership. J Intraven Nurs, 18, 162–4.
Blincyto (R) [package insert]. (2017). Thousand Oaks, CA: Amgen.
IEC (2012, October 19). IEC 60601-2-24:2012. Retrieved from https://www.iecee.org/dyn/www/f?p=106:49:0::::FSP_STD_ID:2635
U.S. Food & Drug Administration. (2017, December 13). What Is an Infusion Pump? Retrieved from https://www.fda.gov/MedicalDevices/ProductsandMedicalProcedures/GeneralHospitalDevicesandSupplies/InfusionPumps/ucm202495.htm
U.S. Food & Drug Administration. (2017, December 31). MAUDE Adverse Event Report: CAREFUSION ALARIS PUMP MODULE PUMP, INFUSION. Retrieved from https://www.accessdata.fda.gov/scripts/cdrh/cfdocs/cfMAUDE/detail.cfm?mdrfoi__id=6431742&pc=FRN
Weinger, M. B., & Kline, A. (2016). Reflections on the Current State of Infusion Therapy. Biomed Instrum Technol. 50(4), 253-262.
About the authors
Ben Powers, BS, MS, is the Vice President of Infusion Systems at Ivenix and Ivyruth Andreica, PharmD, BSN, FISMP, is the Clinical & Medication Safety Pharmacist at Ivenix.